Once operational, wind turbines generate power with virtually no direct emissions, making them a cornerstone of many low-carbon energy strategies. However, it’s important to note that this “cleanliness” starts after the turbines are built.
The production, transportation, and installation of turbines—especially the energy-intensive processes behind manufacturing massive steel towers, fiberglass blades, and rare-earth-based magnets—carry a substantial carbon footprint. Each turbine has a time-to-offset period, often several months to a few years, during which it works to “pay back” its embedded emissions before becoming a net carbon saver.
After this time-to-offset, it does feature lower lifecycle emissions than fossil fuels – if the gearbox actually lasts the 20 years it’s supposed to. And that’s a big if.
15. Global Wind Resources and Ireland’s Potential
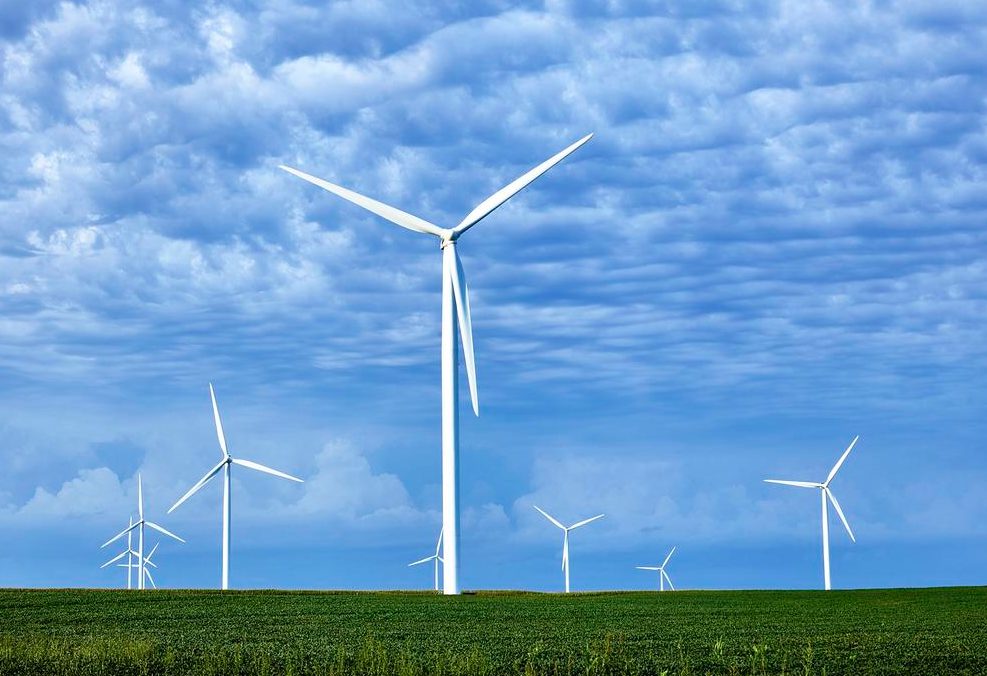
While politicians wax poetic about green energy transitions, the uncomfortable reality of wind power resembles that Netflix series everyone claims to love but few finish watching. Some locations hit the geographical jackpot, others get stuck with the renewable energy equivalent of dial-up internet. The industry’s dirty little secret? Location determines everything.
Ireland’s western coast tells the real story. During optimal wind conditions, turbines can supply up to 70% of the country’s power—still impressive though not the “entire country” claim often recycled in promotional materials. What gets conveniently omitted is how this small island nation’s grid frequently buckles under the unpredictability. Regardless of technical limitations, Ireland continues to invest hundreds of millions annually in new wind capacity, betting that interconnection with mainland Europe will solve its intermittency puzzle.
14. Gearbox Frustrations in Wind Turbines
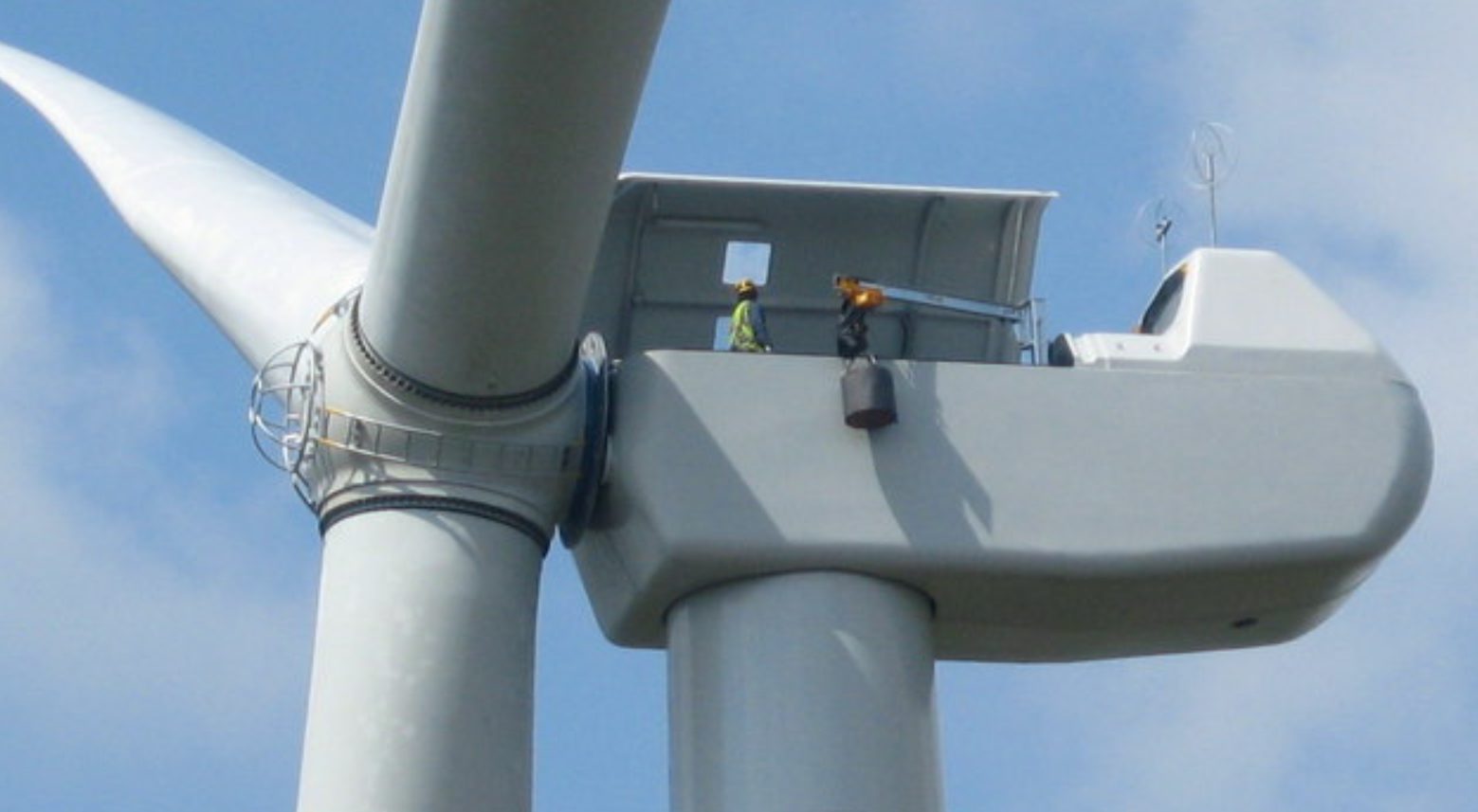
Peek inside a wind turbine’s nacelle and you’ll find the industry’s greatest technical headache: a mechanical Achilles’ heel disguised as engineering brilliance. Every modern turbine depends on massive gearboxes—15-ton mechanical beasts—to transform lazy rotor revolutions into high-speed generator spin. These engineering marvels pack the complexity of a sports car transmission into something the size of your living room.
What manufacturers don’t advertise? These gearboxes frequently implode halfway through their expected lifespans, sending maintenance costs soaring. When offshore installations require specialized vessels costing between $100,000-$250,000 daily just to access failed components, the economics start resembling a casino rather than an investment. Operations and maintenance swallow nearly 20% of total energy costs, creating an entire shadow industry of specialists making their living from this engineered imperfection.
13. Direct Drive Systems and Rare Earth Metals
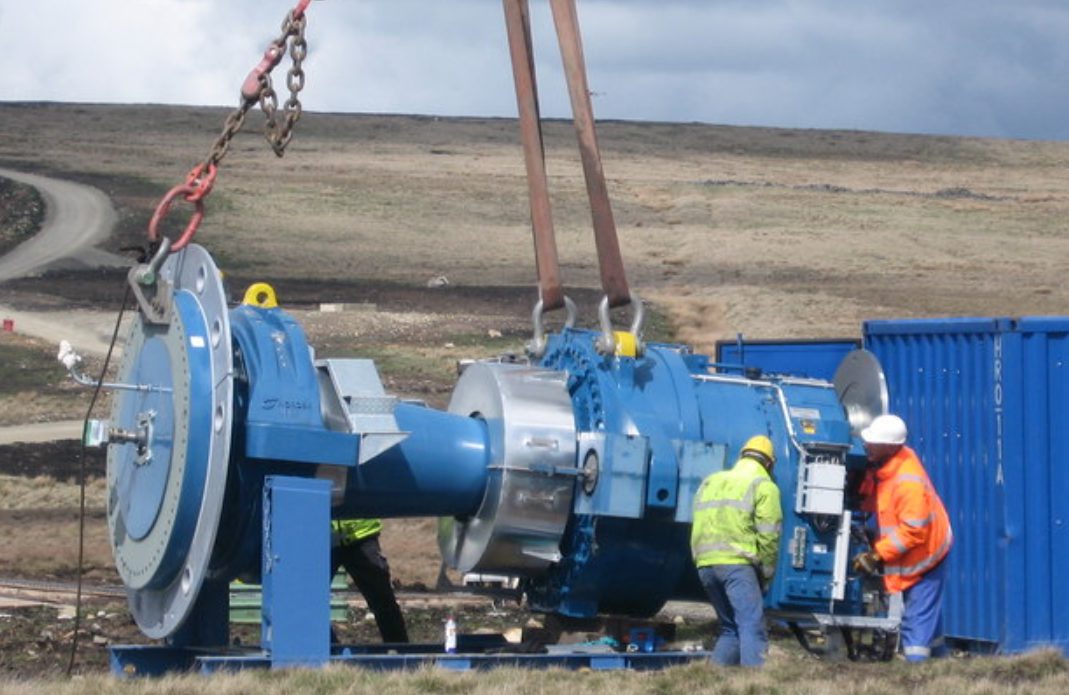
Engineers, tired of watching gearboxes fail spectacularly, developed an elegant solution: eliminate them entirely. Direct drive systems connect massive generators directly to the rotor—like removing the transmission from your car and installing an engine that somehow works at any speed. This architectural simplification reduces moving parts the same way minimalist design cleared your grandparents’ cluttered living room.
Behind this mechanical triumph lurks an inconvenient dependency that energy transition cheerleaders rarely acknowledge. These systems rely on specialized magnets requiring rare earth metals predominantly controlled by China, which currently holds 90% of global supply.
This creates a geopolitical vulnerability about as comfortable as discovering your home’s foundation depends on materials your argumentative neighbor controls exclusively. The environmental damage from rare earth mining remains another carefully cropped element from the pristine wind energy portrait.
12. Maintaining Grid Frequency and Inertia
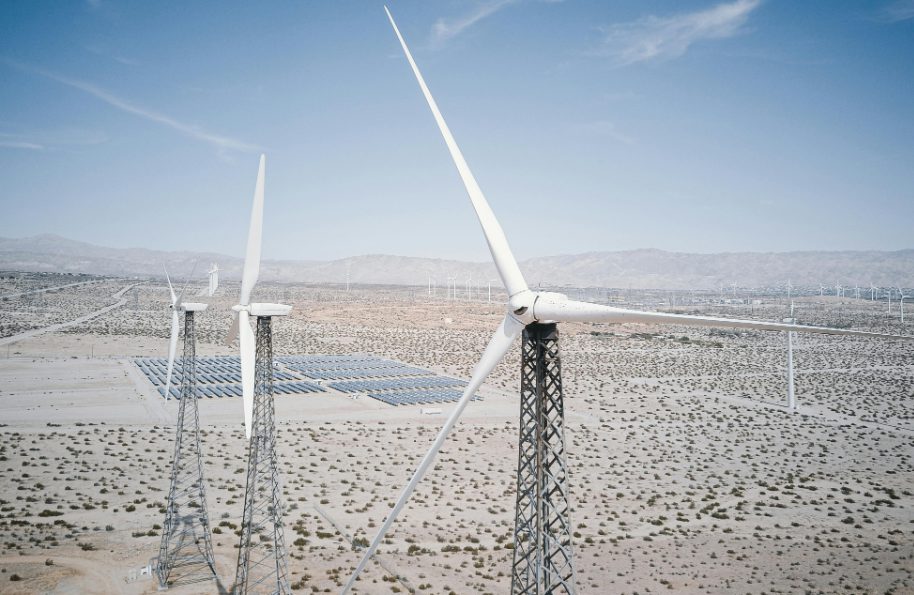
The modern power grid operates like an invisibly choreographed ballet where perfect timing prevents catastrophic failure. Conventional power plants naturally provide “inertia”—a stabilizing physical resistance to change similar to how a spinning top resists falling over. This mechanical property prevents your lights from flickering when your neighbor’s air conditioner kicks on.
Wind turbines connected through computerized inverters contribute virtually zero natural inertia to this delicate system. A deviation of just 1 Hz can cascade into equipment failures reminiscent of dominoes falling—except these dominoes control hospital equipment and water treatment plants. Texas discovered this reality during its 2021 freeze when grid frequency plummeted dangerously close to complete collapse. The industry’s response? A technological arms race developing synthetic substitutes for what traditional generators provided naturally.
11. Variable Speed Wind Turbines and Inverters
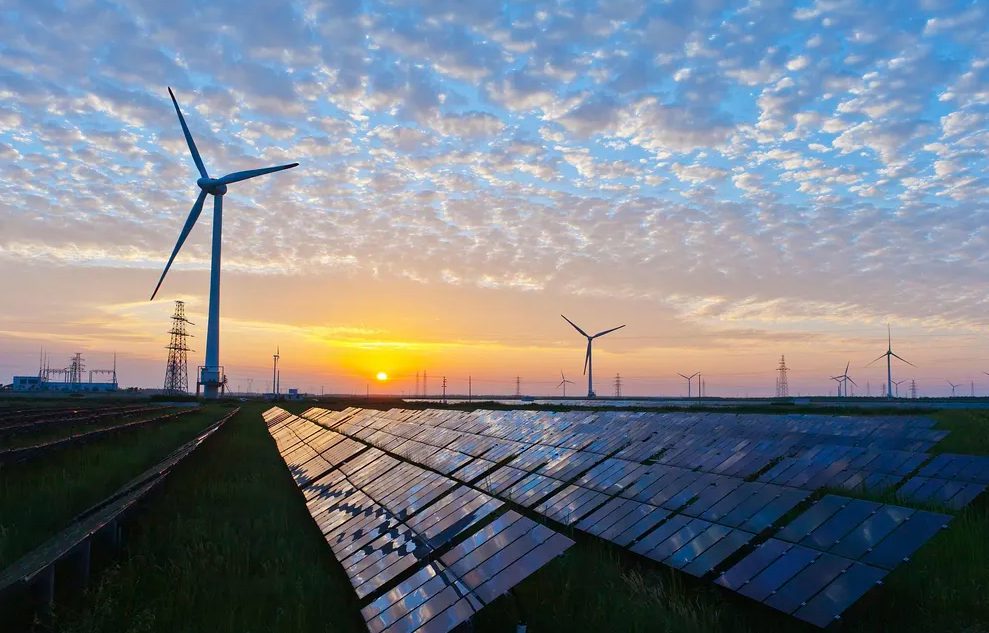
Modern turbines perform a continuous technological tango with changing wind conditions, adjusting rotor speeds moment-by-moment to extract maximum energy. This dance requires sophisticated electronics—specifically inverters—that transform wild, fluctuating electricity into the precise, standardized current your phone charger expects. Without this digital choreography, wind power would remain an interesting science experiment rather than a grid-scale resource.
The electronics enabling this flexibility create their own paradox. They simultaneously boost energy efficiency while further disconnecting turbines from the physical properties that historically stabilized the grid. It’s like replacing a car’s mechanical steering with computerized controls—superior in most situations until the software crashes. These complex systems have become the industry standard not because they’re perfect, but because they’re less imperfect than alternatives. The entire renewable transition hinges on these invisible electronic mediators.
10. Flywheel Technology for Grid Stability
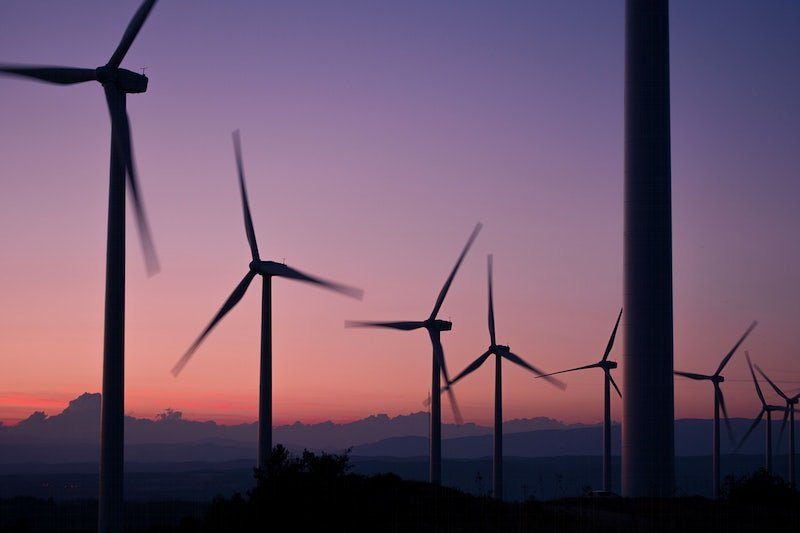
While politicians debate renewable targets from air-conditioned offices, engineers frantically work to prevent those same renewables from destabilizing the grid. Their surprising solution? Massive 120-ton spinning cylinders—essentially industrial-strength fidget spinners—that store energy through pure rotational momentum. These flywheel installations function like mechanical batteries, absorbing or releasing energy within milliseconds to maintain the grid’s heartbeat.
The brilliance lies in repurposing existing infrastructure. Former coal plants—yesterday’s environmental villains—now house these stabilization systems, maintaining grid connection points while transitioning to supporting rather than competing with renewables.
The approach resembles converting an old gas-guzzling muscle car into an electric vehicle charging station. While still limited in deployment compared to lithium-ion batteries, flywheels represent a bridge technology allowing renewables to scale before we’ve fully solved their inherent instability.
9. Interconnections and Hydrogen Economy
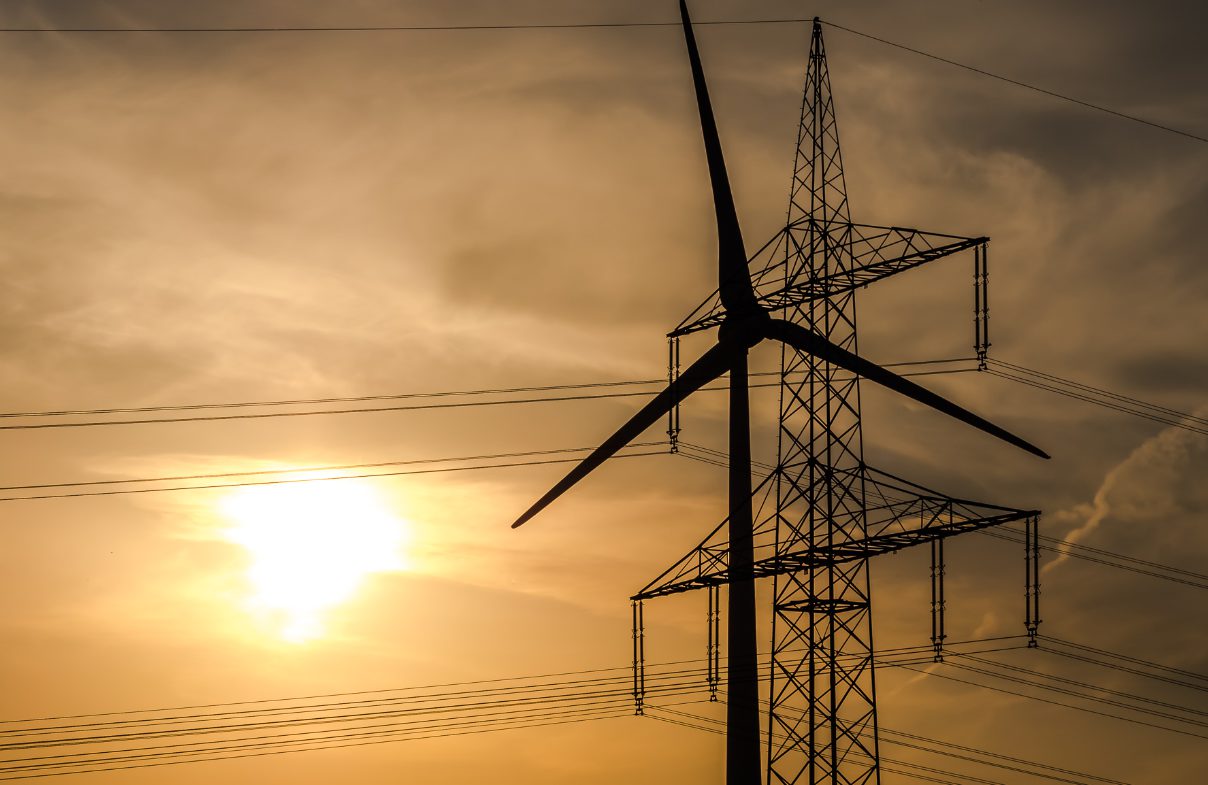
The renewable industry’s unofficial mantra—”the wind’s always blowing somewhere”—drives a massive push for cross-border transmission links that would make 1950s interstate highway planners blush with envy. Ireland exemplifies this strategy with its planned 700 megawatt connection to France, effectively laying a submarine extension cord capable of powering nearly one-fifth of the island’s peak demand. This approach spreads weather risk across continents rather than counties.
Meanwhile, hydrogen emerges as the industry’s Swiss Army knife—a flexible carrier capable of storing surplus wind energy through water-splitting electrolysis. The resulting fuel can power everything from steel mills to container ships, essentially converting intermittent electricity into molecular batteries. Both approaches require investments in billions rather than millions, creating a scale challenge comparable to rebuilding your home’s foundation while still living inside. Despite formidable barriers, these infrastructure bets represent the industry’s preferred path forward.
8. Wind Power’s Cost Effectiveness and Global Potential
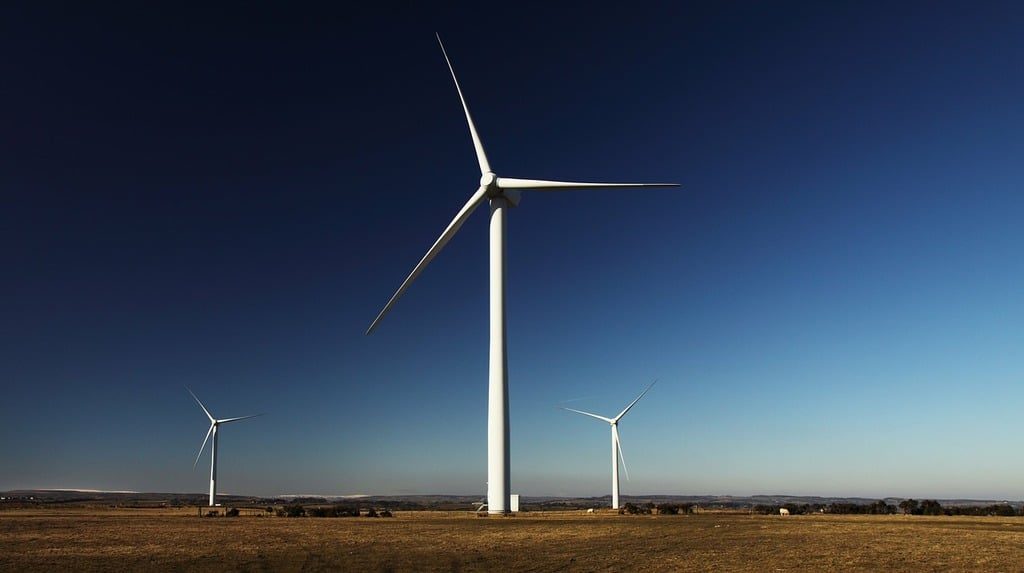
Behind the declining cost charts wind advocates enthusiastically share lies a more complex economic picture resembling an iceberg—with most relevant factors conveniently submerged. The industry correctly notes that wind farms maintain a surprisingly compact footprint, requiring less than one acre permanently per megawatt of capacity. This land efficiency enables agricultural activities to continue largely uninterrupted, creating potential win-win arrangements with rural landowners.
The theoretical potential remains staggering—offshore wind within 60 kilometers of coastlines could technically power modern civilization several times over. This abundance fuels renewable optimism similar to how oil discoveries once triggered petroleum booms. The difference? Wind’s bounty arrives in sporadic, unpredictable bursts rather than on-demand delivery.
The true costs appear in the massive adaptation expenses required throughout the entire electrical system—from transmission to storage to backup generation—that rarely appear in promotional materials.
7. Environmental Impacts of Wind Turbine Construction
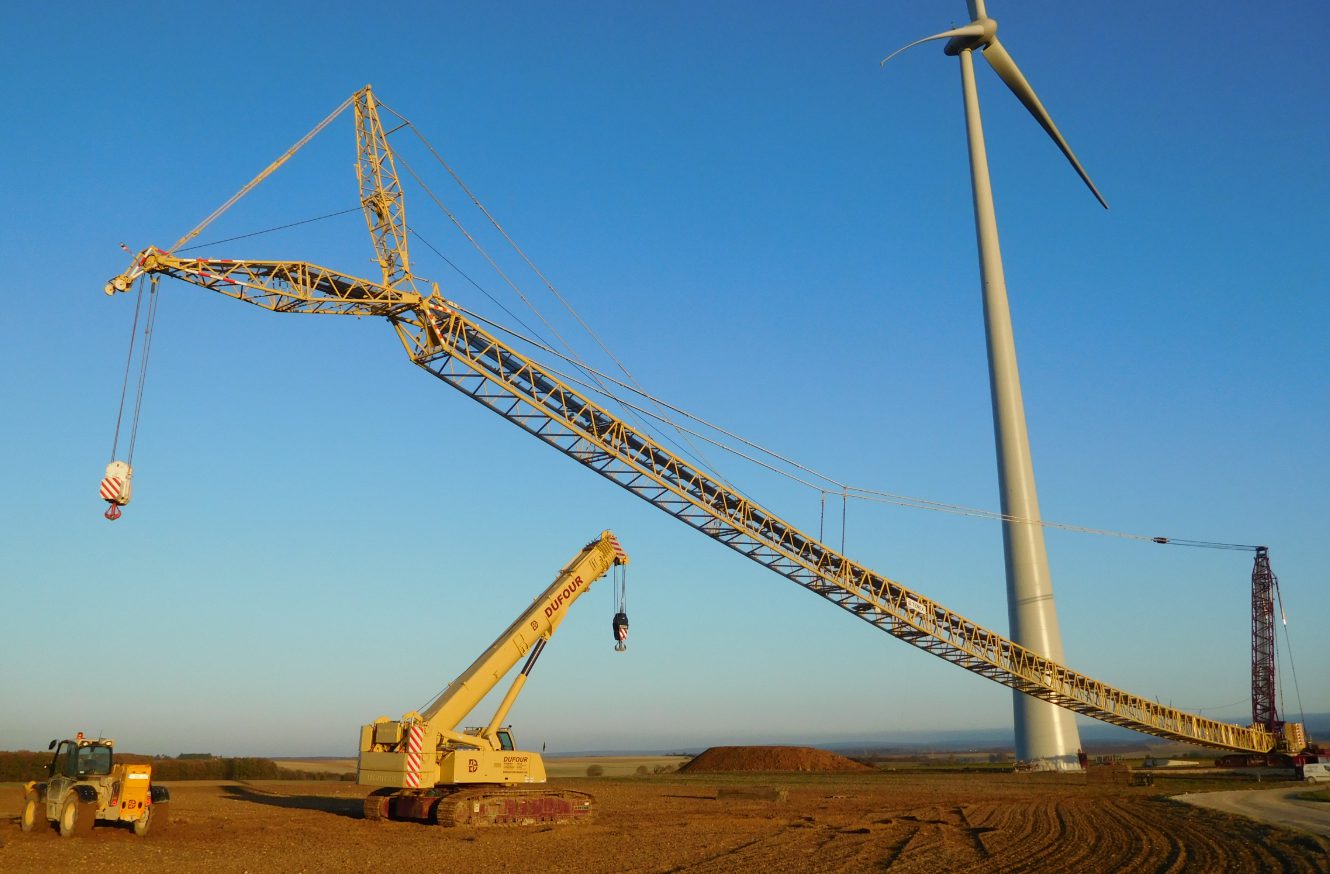
The pristine turbine images adorning corporate websites mask a construction reality closer to heavy industry than eco-friendly development. Each turbine foundation requires a substantial amount of concrete, while access roads carve through previously undisturbed landscapes. Offshore installations require underwater excavation and cable-laying that temporarily disrupt marine habitats like renovations that commandeer an entire house before improving it.
Forward-thinking developers implement damage control strategies throughout project lifespans, routing access roads around sensitive ecosystems and timing construction to avoid wildlife breeding seasons. These mitigation approaches significantly reduce environmental footprints while improving community acceptance. Wind projects maintain lower ongoing impacts compared to fossil alternatives—like comparing a one-time renovation to a perpetually leaking pipe—but the initial ecological disruption remains considerably greater than the postcard-perfect turbine images suggest.
6. Wildlife and Wind Turbine Collisions
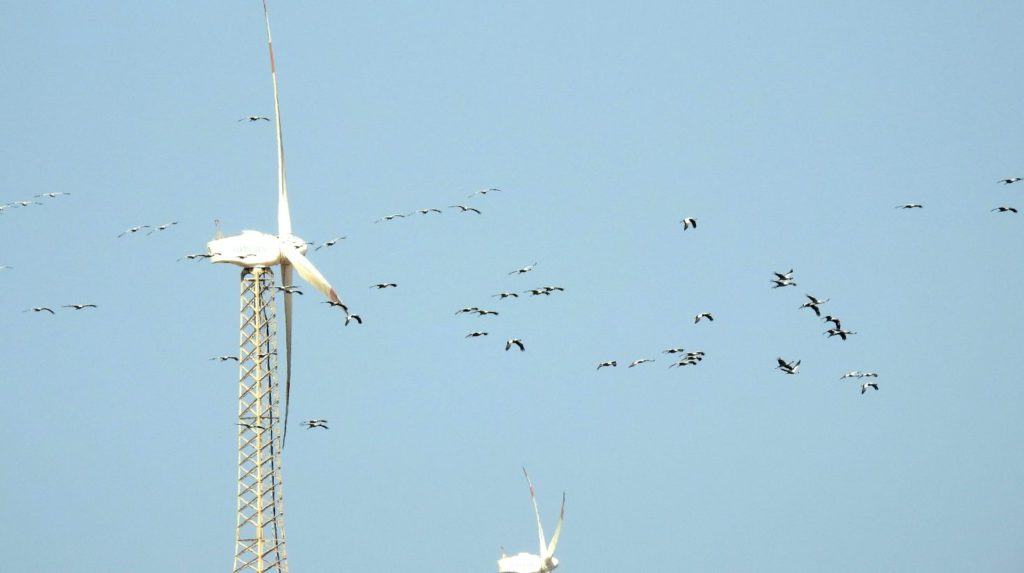
The clean energy narrative collides with ecological reality every time a bird strikes a turbine blade—which happens with disturbing frequency. American turbines cause between 140,000 and 500,000 bird deaths annually, a number expected to climb as installations multiply across migration pathways. Bats fare even worse, susceptible to both direct collisions and the invisible pressure waves that rupture their internal organs like tiny underwater explosions.
Engineers have developed mitigation technologies including radar-activated shutdown systems and blade painting techniques that make turbines more visible to birds—like adding collision warning stripes to glass doors.
Offshore developments create surprising ecological sanctuaries by restricting fishing within arrays, effectively establishing protected marine zones as an unintended consequence. This complex environmental ledger—with clear wildlife costs offset by potential ecosystem benefits—rarely appears in simplified advocacy materials from either supporters or opponents.
5. End-of-Life Turbine Disposal and Recycling Efforts
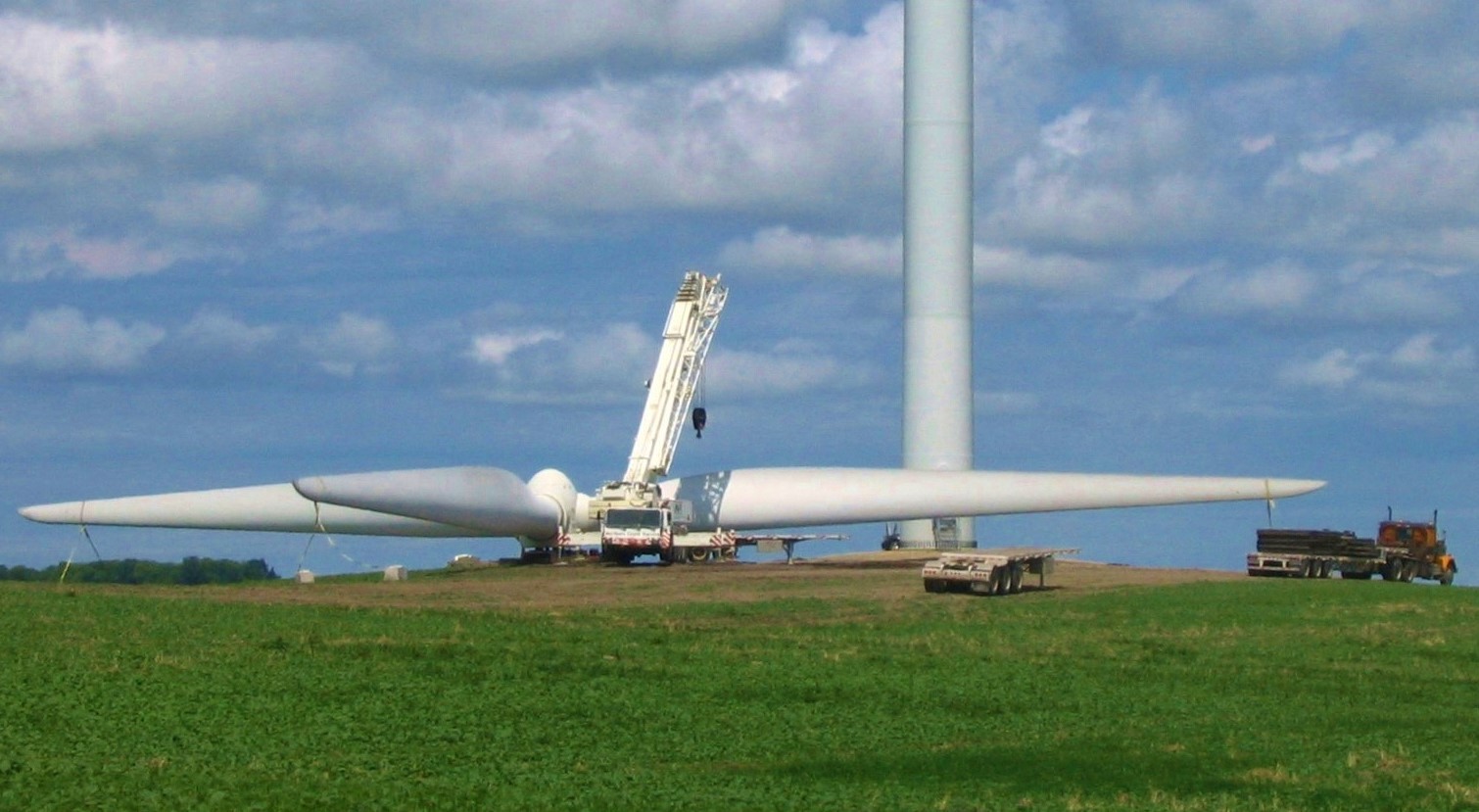
Wind energy’s towering monuments eventually become monumental waste problems that the industry strategically downplays in its marketing materials. Currently, about 85% of turbine components can be recycled through conventional methods—the metals, wiring, and mechanical systems finding ready homes in existing recycling streams. The massive fiberglass blades—some longer than a Boeing 747’s wings—present the stubborn exception to this circular economy narrative.
Few consumers realize the recycling challenges these materials present. Innovative companies have developed promising solutions for blade waste, from grinding them into manufacturing pellets to incorporating them into cement production. These approaches reduce coal consumption in cement kilns while diverting material from landfills. The European regulatory environment increasingly forces this innovation by restricting landfill disposal, creating market-driven solutions through necessity. The entire situation exposes how even the cleanest energy technologies leave waste footprints that require creative engineering to address.
4. Innovations in Wind Turbine Materials and Design
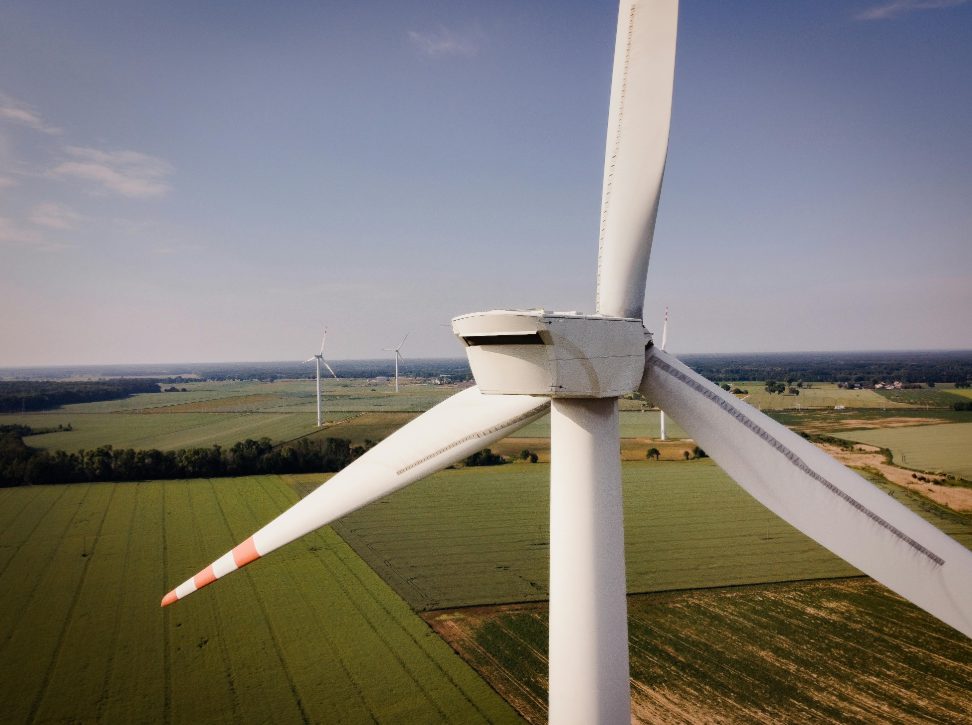
Beyond the monotonous white giants dotting rural landscapes, a design revolution percolates in engineering labs worldwide. Swedish innovators at Modvion have created engineered wood towers that slash embodied carbon dramatically—like switching from concrete to timber framing in home construction. Urban micro-turbines designed for building integration reimagine generation at human scale rather than industrial monuments, potentially unlocking wind energy in previously inaccessible settings.
These innovations face regulatory obstacle courses and durability questions before widespread adoption—similar to how electric vehicles spent decades as interesting prototypes before becoming mainstream products. Sustainability-focused markets and evolving regulations encourage material experimentation across the industry, from recyclable composites to carbon fiber alternatives. The future likely includes diverse approaches tailored to specific environments rather than today’s cookie-cutter deployments, similar to how housing designs vary by climate rather than following a single global template.
3. Life Cycle Analysis and the Future of Wind Energy
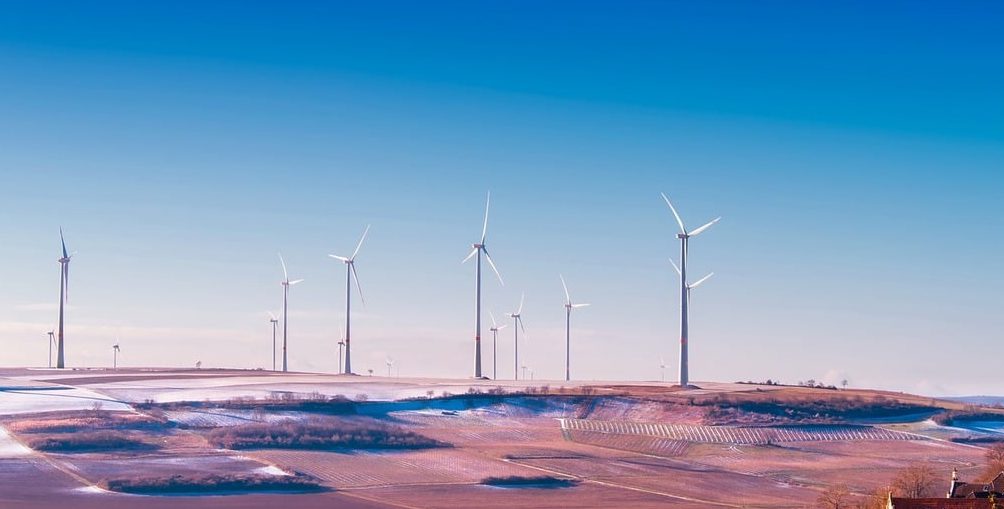
When environmentalists and industrialists actually examine wind energy’s complete life cycle—from mining through manufacturing, operation, and eventual decommissioning—both sides find ammunition for their arguments. Wind consistently ranks among the lowest carbon intensity electricity sources, producing roughly 11 grams of CO2 per kilowatt-hour compared to natural gas at 465g and coal exceeding 980g. This dramatic difference explains why climate-focused analysis overwhelmingly favors wind expansion despite its imperfections.
The analytical approach highlights upstream impacts from materials and manufacturing while demonstrating wind’s overwhelming advantage compared to fossil alternatives—like comparing a one-time mortgage payment to perpetual rent. Manufacturing processes and material selection represent the principal areas for continued improvement, particularly reducing rare earth metal requirements and enhancing component recyclability. As policy frameworks increasingly incorporate full life-cycle emissions into regulatory decisions, wind’s already favorable profile continues improving through innovation.
2. Community Engagement and Socioeconomic Impact
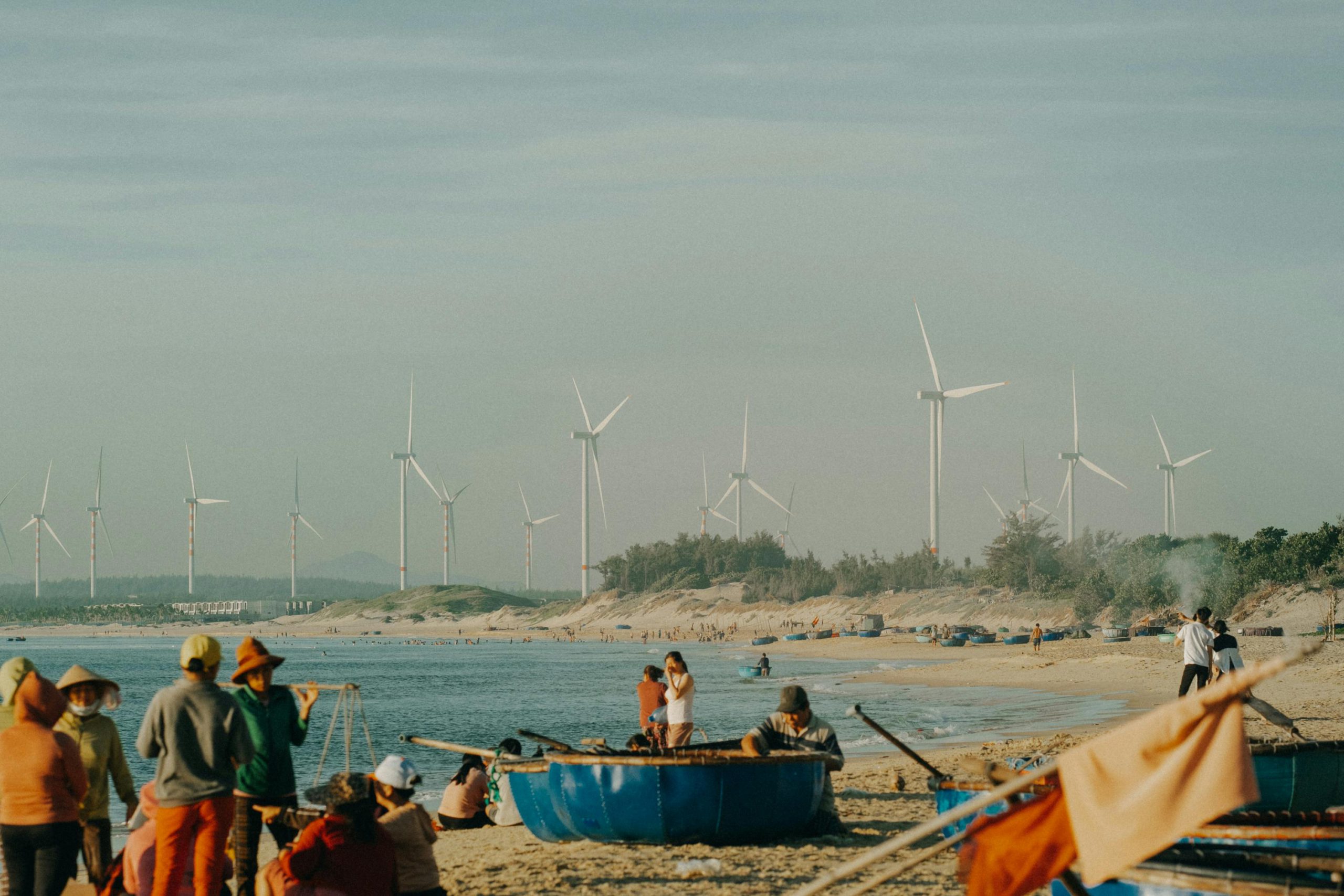
Wind development transforms rural economies like tech companies reshaping urban neighborhoods—for better and worse, depending entirely on who you ask. Projects create employment waves during construction followed by smaller permanent maintenance teams while generating tax revenue streams for previously struggling communities. Landowners hosting turbines receive yearly payments resembling a renewable lottery win—financial security regardless of agricultural market fluctuations.
The economic ripples can be substantial, contributing to a 6.5% increase in per-capita GDP in counties with significant wind development. This prosperity boost appears approximately three times larger in rural areas compared to urban regions, reversing decades of economic decline in some farming communities.
Franklin County, Iowa exemplifies this transformation, where wind energy payments enabled local government to reduce property taxes while maintaining services. When wind energy investments include strong community partnerships, the results often echo the impact of other useful inventions that help communities: boosting local economies and creating lasting social benefits that reach well beyond clean power generation.
1. Hybrid Wind-Solar-Battery Projects
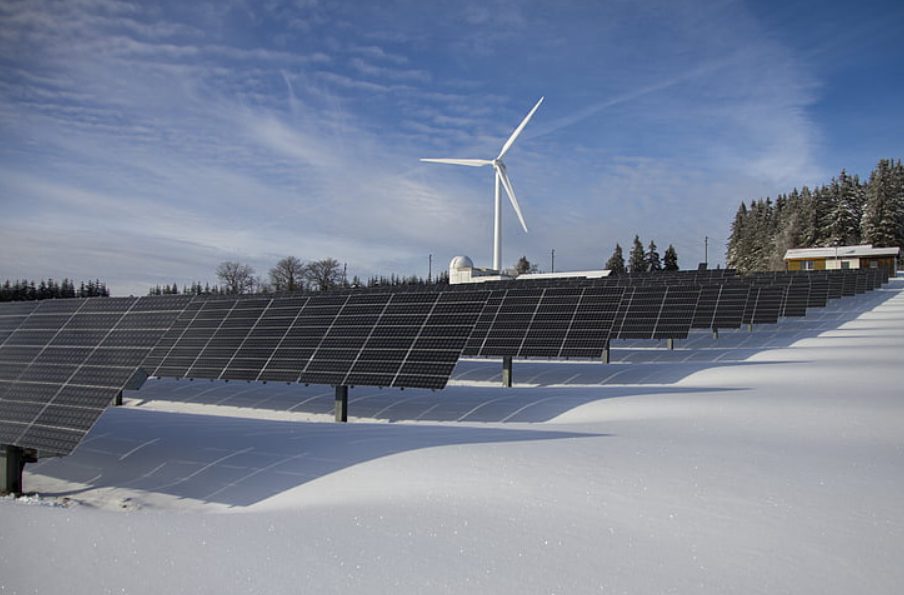
The renewable industry’s latest evolution resembles the smartphone’s transformation from single-purpose device to multi-functional tool. By combining wind turbines, solar panels, and battery systems in unified installations, developers create modern power plants that deliver more consistent output throughout day-night cycles. Advanced software orchestrates this complexity like a technological conductor, balancing the strengths and weaknesses of each generation type.
These integrated facilities maximize transmission infrastructure efficiency—like squeezing multiple apps through a single internet connection—while their battery components buffer variability that would otherwise require fossil fuel backup. This approach substantially reduces conventional backup requirements while enhancing grid stability, addressing wind’s core weaknesses through technological partnership rather than isolated improvement.
Despite higher upfront costs and regulatory complexity, the hybrid model is gaining traction precisely because it solves problems that single-technology approaches cannot. It mirrors many groundbreaking technologies set to transform energy and infrastructure across industries.